Compact Objects and Transients
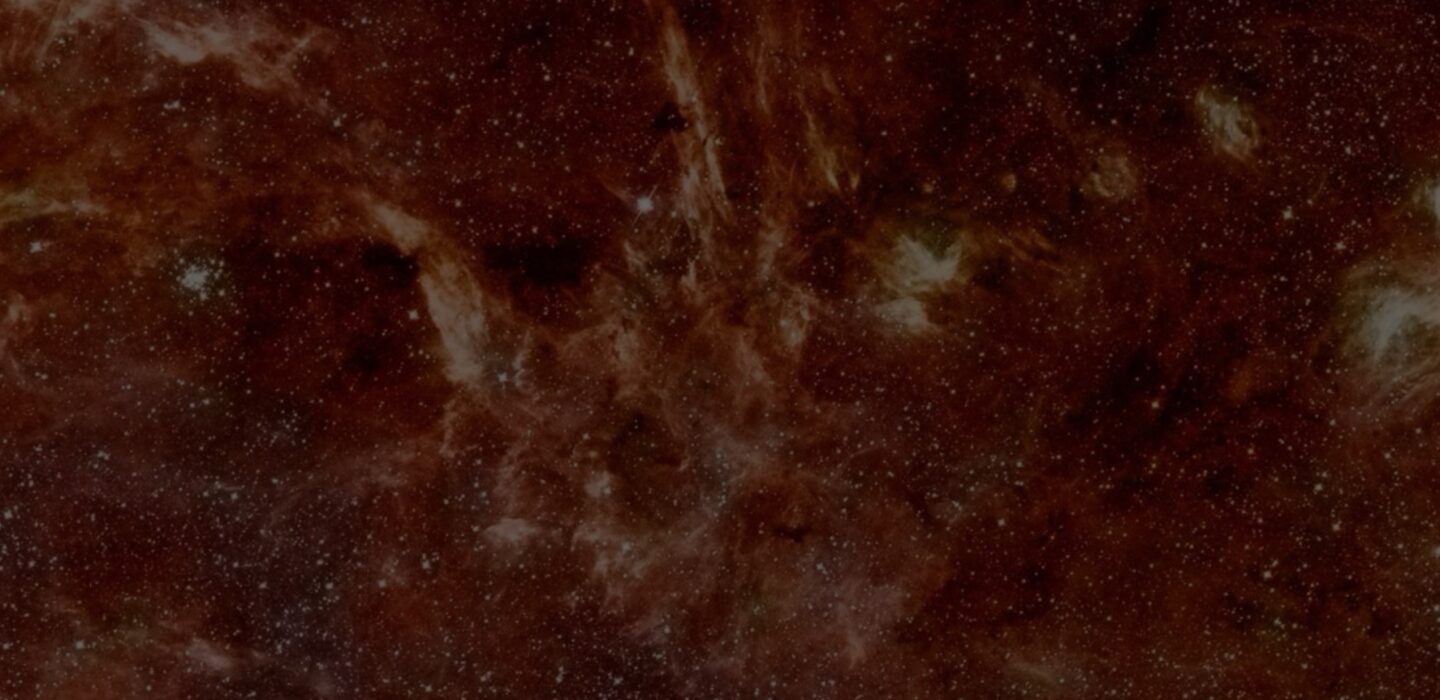
Compact objects (black holes, neutron stars, and white dwarfs) are the final fates of stars, and are amongst the most extreme objects in the known universe, where the laws of physics are pushed to their limits.
For instance, black holes, with their immense gravitational pull, challenge our understanding of space and time and are key to testing Einstein's theory of general relativity. Neutron stars, the densest known objects after black holes, provide insights into the behavior of matter at nuclear densities. White dwarfs, remnants of stars like our sun, contribute to our understanding of stellar evolution and were key in the discovery of the accelerated expansion of our universe.
In recent years, studies of compact objects have opened a new window thanks to the detection of gravitational waves from colliding black holes or neutron stars with LIGO. Thus, the study of compact objects not only enriches our knowledge of the cosmos but also drives advancements in fundamental physics.
MKI researchers are studying compact objects using a wide range of facilities. For instance, the MIT LIGO group regularly detects the mergers of compact objects via gravitational wave emission; MIT developed the detectors on the Neutron Star Interior Composition Explorer on the International Space Station, that uses X-ray timing to measure the neutron star equation of state. The Chandra High-Energy Transmission Grating Spectrometer, built at MIT, continues to observe signatures of accretion of gas on to compact objects, e.g. from black holes with stellar companions. All of these facilities build a rich multi-messenger tool-kit to understand the most extreme objects in the universe.
Black hole accretion
There are millions of unknown stellar mass black holes lurking in our own galaxy, and yet, only of order 150 of these are known because they exist as part of a binary. X-rays arising from material from the star accreting onto the black hole is one way to reveal the black hole(s). They can become the brightest sources in the X-ray sky. In recent years, there has been important progress in our understanding of black hole X-ray binaries thanks to X-ray Reverberation Mapping, a technique advanced by Prof. Erin Kara and her research group over the past decade, where X-rays produced close to the black hole reverberate off inflowing gas. This allows them to map scales close to the event horizon—well beyond the resolution of any telescope. Thanks to these new techniques and the launch of the NICER Observatory with its unprecedented throughput and time resolution, astronomers now understand the accretion flow geometry well enough to accurately measure spins in stellar-mass black holes. This is especially important now that LIGO regularly detects binary black holes. Using mass and spin distributions of these different populations is essential for constraining binary evolution scenarios.
Accreting neutron stars
The work of Prof. Deepto Chakrabarty’s group focuses on studies of accreting neutron stars, often using the Neutron Star Interior Composition Explorer (NICER), an x-ray telescope on the International Space Station, and the Nuclear Spectroscopic Telescope Array (NuSTAR). This work includes studies of thermonuclear detonations of accreted material and studies of x-ray pulsars. Recent work has provided strong constraints on the radii of several neutron stars, placing strong constraints on the equation of state of dense nuclear matter.
Compact object mergers via gravitational waves
Many compact objects – luminous ordinary stars, neutron stars, and black holes – are in binary systems. In the end phase, the two objects inspiral with such speed that they make measurable gravitational waves. The research group around Dr. Erik Katsavounidis, Prof. Scott Hughes, Dr. David Shoemaker and Prof. Salvo Vitale are focusing on these waves and to interpret them within the framework of general relativity. The results give detailed information about the two objects – their distance, mass, inclination of orbit, and spins; they can be considered “standard sirens” in analogy to “standard candles” used in electromagnetic observations. With the data for populations and individual objects, the distribution in space, in mass, in spin, and other parameters can be extracted. Searches for exotic sources, such as supernovae, sub-solar mass systems, and cosmic strings are underway. Spinning neutron stars with even millimeter-scale asymmetries over their ~10 km radius will radiate measurable gravitational waves, and will reveal information about nuclear matter when detected. The most spectacular possibilities are for multi-messenger astrophysics with gravitational waves, electromagnetic, and particle observations. In 2017, a first such event took place (named GW170817), and others will shed light on the many properties of the compact objects involved. Future gravitational-wave detectors with MKI involvement will extend our reach to the edge of the universe, for stellar-mass compact objects: MKI-Led Cosmic Explorer, and LISA, a space-based detector for intermediate- and supermassive black holes
Populations of gravitational-wave sources
Prof. Salvo Vitale’s research group focuses on the astrophysical interpretation of the gravitational-wave sources detected by LIGO. In what astrophysical environment did these black holes and neutron stars form? What were the properties of their progenitor stars? These are all questions that can be addressed with the information encoded in an object’s detected gravitational wave signature. Prof. Vitale’s group is also exploring the scientific potential of the next generation gravitational-wave detector concept “Cosmic Explorer”, which will be online in the 2030s and will detect black holes merging when the universe was only a few tens of million years old.
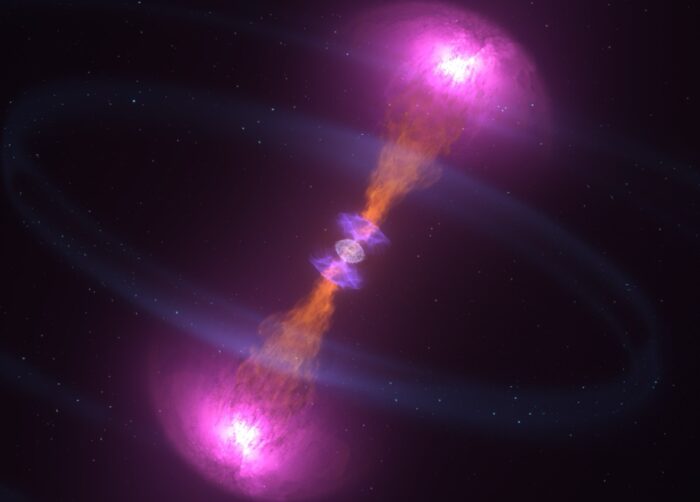
An artistic simulation of GW170817, showing gravitational-waves spiraling out in the plane of the inspiral, and neutron ejecta, and electromagnetic waves as jets perpendicular to the plane.
Credit: NASA's Goddard Space Flight Center/CI Lab