Alex Schekochihin
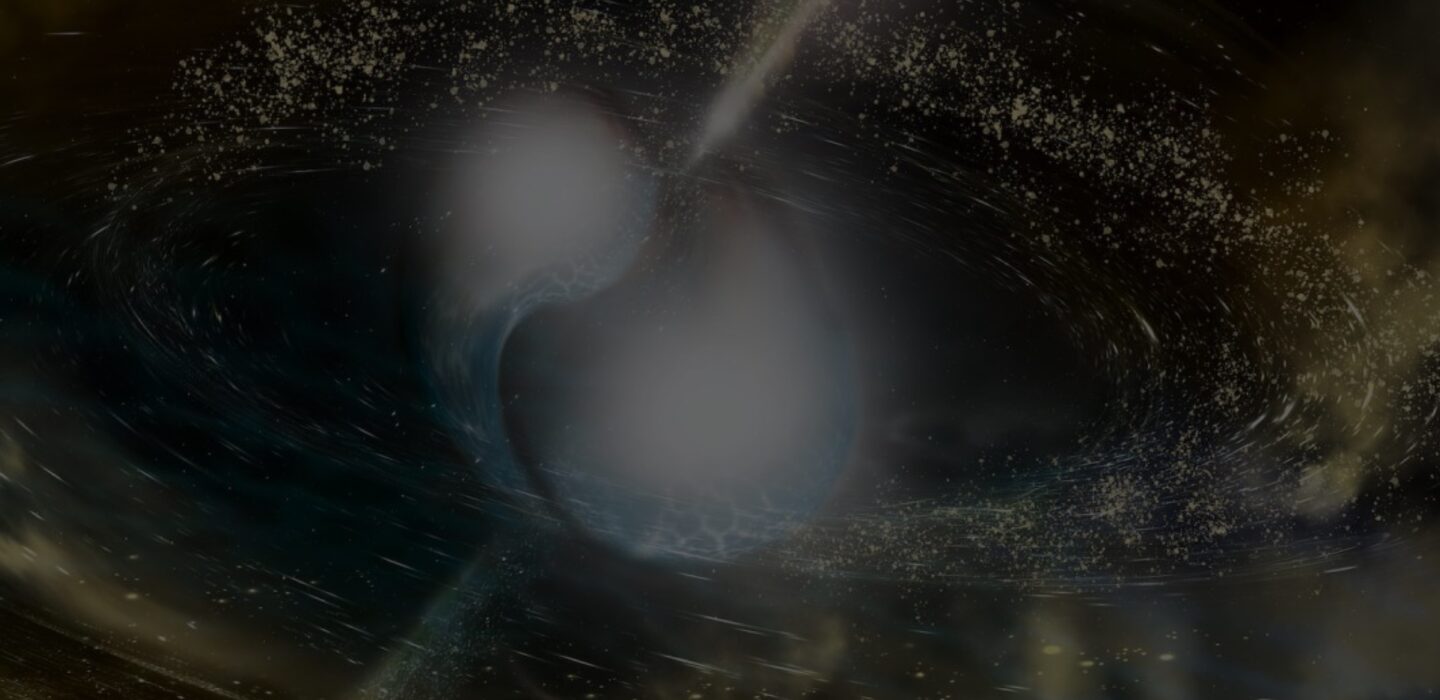
Tuesday, September 21 2021
4:00pm
only via zoom
A modeler’s approach to visible (and, indeed, also dark) matter in the Universe is to think of it as a fluid, write some version of (magneto)hydrodynamical equations for its density, velocity and temperature, code them up and model away. By and large, this has given us a successful (at least visually) description of many astrophysical objects made of plasma: fluid-dynamical fictions tend to look quite reamrkably like observed reality on large scales. Some difficulties arise when one wants to know how the large-scale coherent energy of motions and fields is “thermalized”, i.e., converted into the internal energy of particles. In theorizing about this topic, we have largely stuck to the paradigms inspired by conventional thermodynamics, based on the notion that the plasmas of interest feature an underlying (local) Maxwellian equilibrium, making concepts such as temperature, entropy, heating, free energy, etc. specific and meaningful. All the dynamics (usually turbulent) are then contained in the first three moments of the local Maxwellian and the departures from this distribution remain small – even in kinetic approaches when, despite being small, they matter. There has been some great progress in understanding such systems theoretically and in matching this understanding to observations at both system-size and small (turbulence) scales – from intergalactic medium [1,2] to the solar wind [3]. Much work in this vein is ongoing and not only prodigious quantities of incremental research but also conceptual advances are in the offing. I would like, however, to discuss what I believe to be the next frontier for plasma theoreticians and attempt to grapple with the fact that many astrophysical plasmas (solar wind being the most accessible of them) are too collisionless (certainly on smallish scales) to be Maxwellian. In solar wind, non-Maxwellian distributions are reported routinely, and PIC codes pick them up quite clearly, although predicting/explaining them is often difficult. How then do we construct a thermodynamics of such a plasma? This is a question that was much discussed in the 1960s and 70s but somewhat fell into abeyance with the rise of computational fluid dynamics. It is now coming back into fashion, with the rise of computational plasma kinetics (in the adjacent area of galactic dynamics, astrophysical theorists have always been interested in non-Maxwellian equilibria, or lack of equilibria, and so that field, which initially trailed behind plasma kinetics, has been leaping ahead of it in some very exciting ways). So I will pick up where the sages of old left off and discuss a number of very simple “undergraduate” questions, the answers to all of which are, however, still extremely tentative, or wholly unknown:
- In a collisionless plasma, how to define the free energy of a distribution?
- How to estimate how much of this energy can be extracted from an unstable distribution and put into electromagnetic fields (usually turbulent)?
- Are there universal collisionless equilibria towards which this turbulence will push the plasma?
- What do we need to know about the properties of this turbulence to work out how plasma relaxes towards those equilibria?
This talk should be suitable for a general physics audience from the late undergraduate level up. Some of the new results that I will talk about are yet to be published (or indeed to become publishable), but the background and the key ideas (although not quite in the order in which I will present them) can be read up on in these lecture notes (§§9-11; updates posted in real time). A discussion of some curious properties of phase-space turbulence can be found in [4,5] (in general theory terms) and [6] (numerics in application to density turbulence in the solar-wind plasma).
[1] I. Zhuravleva et al., Nature Astron. 3, 832 (2019) [e-print arXiv:1906.06346]
[2] I. Zhuravleva et al., Nature 515, 85 (2014) [e-print arXiv:1410.6485]
[3] J. Squire et al., submitted (2021) [e-print arXiv:2109.03255]
[4] T. Adkins and A. A. Schekochihin, J. Plasma Phys. 84, 905840107 (2018) [e-print arXiv:1709.03203]
[5] A. A. Schekochihin et al., J. Plasma Phys. 82, 905820212 (2016) [e-print arXiv:1508.05988]
[6] R. Meyrand et al., Proc. Nat. Acad. Sci. 116, 1185 (2019) [e-print arXiv:1808.04284]
Speaker
- Alex Schekochihin, Rudolf Peierls Center for Theoretical Physics, University of Oxford
Host
Event Contact
- Debbie Meinbresse